Here are some stem cell stories that caught our eye this past week. Some are groundbreaking science, others are of personal interest to us, and still others are just fun.
Stem cells heal diabetic foot ulcers in pilot study
Foot ulcers are one of the many long-term complications that diabetics face. About 15 percent of patients develop these open sores which typically appear at the bottom of the foot. In a quarter of these cases, the ulcers lead to serious infection requiring amputation.

Diabetic foot ulcers are open sores that don’t heal and in many cases leads to amputation. Image source: Izunpharma
But help may be on the horizon in the form of stem cells. Researchers at Mansoura University in Egypt recently presented results of a small study in which 10 patients with diabetic foot ulcers received standard care and another 10 patients received standard care plus injections of mesenchymal stem cells that had been collected from each patient’s own bone marrow. After just six weeks, the stem cell treated group showed a 50% reduction in the foot ulcers while the group with only standard care had a mere 7% reduction.
These superior results with the stem cells were observed even though the group receiving the stem cells had larger foot ulcers to begin with compared to the untreated patients. There are many examples of mesenchymal stem cells’ healing power which make them an extremely popular cell source for hundreds of on-going clinical trials. Mesenchymal stem cells are known to reduce inflammation and increase blood vessel formation, two properties that may be at work to give diabetic foot ulcers the chance to get better.
Medscape Medical News reported on these results which were presented at the 2016 annual meeting of the European Association for the Study of Diabetes (EASD) 2016 Annual Meeting
Suppressing nerve signals to help spinal cord injury victims
Losing the use of one’s limbs is a profound life-altering change for spinal cord injury victims. But their quality of life also suffers tremendously from the loss of bladder control and chronic pain sensations. So much so, victims often say that just improving these secondary symptoms would make a huge improvement in their lives.
While current stem cell-based clinical trials, like the CIRM-funded Asterias study, aim to reverse paralysis by restoring loss nerve signals, recent CIRM-funded animal data published in Cell Stem Cell from UC San Francisco suggest that nerve cells that naturally suppress nerve signals may be helpful for these other symptoms of spinal cord injury.
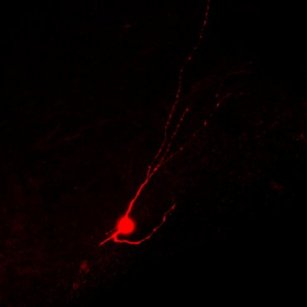
Mature inhibitory neuron derived from human embryonic stem cells is shown after successfully migrated and integrated into the injured mouse spinal cord.
Photo by Jiadong Chen, UCSF
It turns out that the bladder control loss and chronic pain may be due to overactive nerve signals. So the lab of Arnold Kriegstein transplanted inhibitory nerve cells – derived from human embryonic stem cells – into mice with spinal cord injuries. The scientists observed that these human inhibitory nerve cells, or interneurons, successfully made working connections in the damaged mouse spinal cords. The rewiring introduced by these interneurons also led to reduced pain behaviors in the mice as well as improvements in bladder control.
In a Yahoo Finance interview, Kreigstein told reporters he’s eager to push forward with these intriguing results:
Arnold Kriegstein, UCSF
“As a clinician, I’m very aware of the urgency that’s felt among patients who are often very desperate for treatment. As a result, we’re very interested in accelerating this work toward clinical trials as soon as possible, but there are many steps along the way. We have to demonstrate that this is safe, as well as replicating it in other animals. This involves scaling up the production of these human interneurons in a way that would be compatible with a clinical product.”
Expanding the CRISPR toolbox
If science had a fashion week, the relatively new gene editing technology called CRISPR/Cas9 would be sure to dominate the runway. You can think of CRISPR/Cas9 as a protein and RNA complex that acts as a molecular scissor which directly targets and cuts specific sequences of DNA in the human genome. Scientists are using CRISPR/Cas9 to develop innovative biomedical techniques such as removing disease-causing mutations in stem cells in hopes of developing potential treatments for patients suffering from diseases that have no cures.
What’s particularly interesting about the CRISPR/Cas9 system is that the Cas9 protein responsible for cutting DNA is part of a family of CRISPR associated proteins (Cas) that have similar but slightly different functions. Scientists are currently expanding the CRISPR toolbox by exploring the functions of other CRISPR associated proteins for gene editing applications.
A CIRM-funded team at UC Berkeley is particularly interested in a CRISPR protein called C2c2, which is different from Cas9 in that it targets and cuts RNA rather than DNA. Led by Berkeley professor Jennifer Doudna, the team discovered that the CRISPR/C2c2 complex has not just one, but two, distinct ways that it cuts RNA. Their findings were published this week in the journal Nature.
The first way involves creation: C2c2 helps make the guide RNAs that are used to find the RNA molecules that it wants to cut. The second way involves destruction: after the CRISPR/C2c2 complex finds it’s RNAs of choice, C2c2 can then cut and destroy the RNAs.
Doudna commented on the potential applications for this newly added CRISPR tool in a Berkeley News release:
Jennifer Doudna: Photo courtesy of iPSCell.com
“This study expands our molecular understanding of C2c2 to guide RNA processing and provides the first application of this novel RNase. C2c2 is essentially a self-arming sentinel that attacks all RNAs upon detecting its target. This activity can be harnessed as an auto-amplifying detector that may be useful as a low-cost diagnostic.”